Spectroscopic Analysis of Benzocaine Using 1H NMR, 13C NMR, MS, and IR Spectroscopy
Introduction
Benzocaine is a commercially available drug that belongs to the amino ester class of local anaesthetic drugs (Singh, et al., 2024). It is used in a range of settings, such as the management of minor traumas, preparation for infiltrative anaesthesia, and dental procedures. Topical application of benzocaine relieves/reduces painful stimuli, such as the ones associated with the needle penetration. As a result, the use of this drug allows for sophisticated pain control along with the reduction of the patient’s anxiety. Tablets, liquids, and benzocaine gels are approved by the FDA (Food and Drug Administration); however, spray-based formulations did not receive the necessary approval and were recalled due to the presence of benzene (Gondim, et al., 2018). Looking at specific applications, benzocaine is heavily used for the relief of pharyngitis-caused pain. Nevertheless, all benzocaine applications should be done with caution due to the possibility of methemoglobinemia as a side effect (Chrubasik, et al., 2012).
The chemical structure of benzocaine is outlined below:
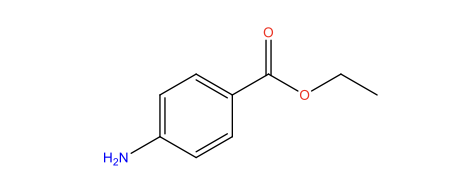
Figure 1: Benzocaine, adapted from (DrugBank, 2024)
The drug performs its functions by reversibly interacting with and inhibiting the activity of sodium channels located in the membrane of neuron cells. Benzocaine enters the respective cells in its nonionised form and subsequently becomes ionised upon passing through the membrane barrier. The corresponding ionised form of benzocaine binds the alpha-subunit, reducing the activity of voltage-gated sodium channels. As a result of the binding, cellular depolarisation is stopped, and the propagation of signals is reduced, while at the same time decreasing the ability of the action potential to form. Local anaesthetics, including benzocaine, can easily form bonds with sodium channels that are in the open configuration. This property is explained by the relatively low pKa of the drug (2.6) compared to other drugs that perform similar functions (Lirk, et al., 2014).
Considering drug pharmacokinetics, it should be indicated that the presence of the aromatic ring is critical for the drug absorption in lipids, enabling the diffusion process across the membranes. The distribution of benzocaine is dictated by the tissue mass, aligning with the blood/tissue partition coefficient. Drug metabolism is supported by pseudocholinesterase, promoting the conversion of benzocaine into para-aminobenzoic acid with its following elimination along with the unreacted benzocaine through the kidneys (DrugBank, 2024).
The aim of the work is to carry out a laboratory experiment leading to the synthesis/purification of benzocaine and analyse its structure using 1H NMR, 13C NMR, MS, and IR Spectroscopy.
Materials and methods
General information
Unless stated otherwise all materials, solvents, and reagents were obtained from commercial sources and used with no additional purification. Analytical grade solvents were used to support the reactions (>99.9%) with manipulations in acetone-washed and flame-dried glassware. A Bruker Avance III HD NMR Spectrometer was used for 1H NMR and 13C NMR. This apparatus is able to obtain both proton- and carbon-NMR spectroscopy at various magnetic strengths. Deuterated chloroform was used as a solvent for both proton and carbon NMR. Infrared (IR) spectroscopy was carried out using the PerkinElmer Spectrum Two FT-IR Spectrometer apparatus, while mass spectrometry (MS) data was collected with the aid of the Agilent 6500 Series Q-TOF LC/MS machine.
Benzocaine synthesis
Benzocaine was synthesised according to the following reaction scheme:

Figure 2: Benzocaine synthesis, adapted from (Saadat, et al., 2023)
p-aminobenzoic acid (0.360g) was placed with ethanol (3.6mL) in a conical flask (10 mL). The mixture was stirred and dissolved until a uniform formation was produced. With stirring, concentrated sulphuric acid (0.30mL) was added dropwise. The introduction of sulphuric acid caused the formation of the hydrogen sulfate salt precipitate of p-aminobenzoic acid, but this precipitate was dissolved during the following reflux stage, during which the hydrogen sulfate salt of the acid was converted into the respective hydrogen sulfate salt of the ester. In order to prepare the reflux conditions, a water-cooled condenser was attached to the flask. The contents of the flask were heated to the boiling point and stirred for 80 minutes. The progression of the reaction was monitored using TLC. When the reaction was completed, the apparatus was disassembled and the mixture was allowed to cool. The solution was transferred to a small Erlenmeyer flask that contained approximately 3mL of deionised water. The reaction mixture completely dissolved in water because the ethyl p-aminobenzoate was in the form of hydrogen salt. When the mixture cooled to the ambient temperature, a solution of sodium carbonate (10%) was added dropwise to completely neutralise the reaction mixture. Sodium carbonate was added until the gas formation was stopped and the pH reached 8. The outlined procedure resulted in a complete neutralisation of sulfuric acid and the formation of the ethyl p-aminobenzoate precipitate. The product was collected, washed with water, and dried overnight (m=0.37g; Yield=85%). The melting point of the product was determined to be 920C, which confirmed its purity.
Results and discussion
Mass Spectrometry (MS) analysis
Mass spectrometry is a highly powerful analytical tool that can be used to characterise a considerable number of organic compounds (Clayden, et al., 2012). This technique is commonly used during the analysis of new compounds in laboratory practice. The characterisation process presumes the conversion of the analysed compound in the form of gaseous ions. This process can be carried out with or without fragmentation. The generated ions are subsequently characterised by the mass-to-charge ratios (m/s) as well as their relative abundance. In turn, free radicals, which can also form as a result of fragmentation, remain undetected. Summarising the above, the purpose of the technique is to characterise the compound based on the effect of the ionising energy on its molecular structure (Clayden, et al., 2012).
Major components of the instrument included the source of ions, the analyser, and the detection system. The purpose of the ion source is to generate a stream of ions from the analysed substance. In turn, the analyser resolves the ions into their respective mass components based on the produced mass-to-charge ratios. Finally, the detector system, confirms the presence of ions as well as determines their relative abundance of the resolved ionic species (Clayden, et al., 2012).
The mass spectrum of the synthesised benzocaine is outlined in the figure below:
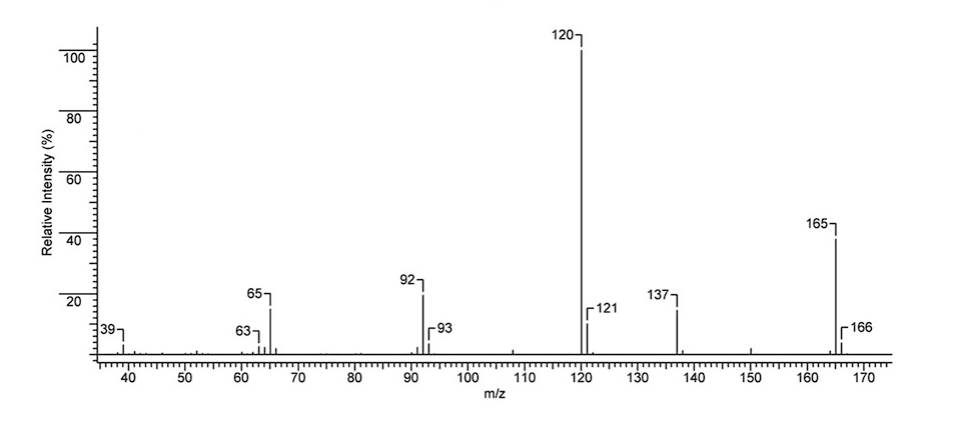
Figure 3: Benzocaine electron ionisation mass spectrum experimental data
As it can be seen in the experimental mass spectrum data above, the top MS peaks are at 65.0, 92.0, 120.0, 137.0, 165.0. The intensities of the indicated peaks are 23.43%, 29.62%, 99.99%, 16.87%, and 25.30%. The indicated peaks can be associated with the formation of the following ions: C5H5+ (65.0), C6H6N+ (92.0), C7H6NO+ (120.0), C7H7NO2+ (137). The peak at 165 is the molecular peak corresponding to the molecular mass of the analysed compound (C9H11NO2). The mass spectrum data also indicated that in addition to the described 5 major peaks, a range of minor peaks were also present. These peaks corresponded to minor charged fragments that can also form during ionisation. For instance, the peak at 121 can be associated with the presence of a 13C carbon in C7H6NO+, which moves the signal from 120 to 121. A similar explanation is valid in other cases (93, 166); however, a different ionisation path is also possible. For example, a minor peak at 138 may be explained by the formation of a C7H8NO2+ ion (Analysis based on (Clayden, et al., 2012)).
1H NMR Spectroscopy analysis
Proton NMR spectroscopy is a highly versatile and straightforward method for analysing the dominating number of organic compounds (Claridge, 2016). In combination with other analysis methods, 1H NMR is heavily used to confirm the structure of new compounds by proving the most critical information regarding the 3D rearrangement of hydrogen atoms in the new molecule. The corresponding NMR spectrum is obtained by sweeping or changing the magnetic field over a small range while observing the radio frequency signal emitted from the sample. At the same time, it is also possible to change the radio frequency of the radiation while maintaining the constant external field (Claridge, 2016).
Benzocaine 1H NMR spectroscopy data is presented in the figure 4:
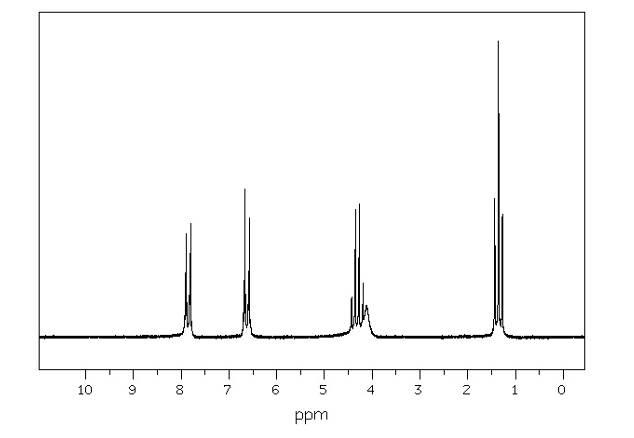
Figure 4: Benzocaine 1H NMR (600 MHz CDCl3) Proton peaks outlined in Figure 4 were assigned to benzocaine in the following fashion:
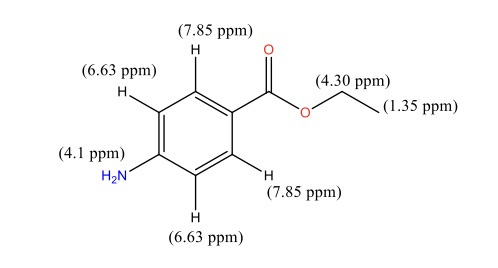
Figure 5: Benzocaine 1H NMR peak assignment
Discussing the proton peaks outlined in Figure 5, it should be indicated that although the structure of benzocaine is relatively complex, all the peaks are clearly visible. In particular, the first peak that appears in the upfield area of 1.35 ppm is -CH3 of the ethyl group. These protons are the most heavily shielded from electromagnetic radiation and, for this reason, appear in the most upfield area. CH3 protons also appear in the form of a triplet due to the coupling with the adjacent -CH2– protons. Conversely, -CH2– ethyl protons were identified at 4.30 ppm. They are less shielded than -CH3 protons and, for this reason, were expected to appear in a more downfield area. In the majority of cases, alkyl protons appear in the area of 1-2 ppm, but the presence of an electron-withdrawing -CO-O- group pushes the indicated protons downfield to 4.30 ppm. -CH2– protons appear as a quadruplet due to coupling with the -CH3 protons. As indicated in Figure 4, the -CH2– protons signals overlap with a broad singlet of -NH2, which appears in its expected area of 4-5 ppm. The peak is observed as a singlet due to the absence of nearby protons. Finally, the remaining two types of protons of the benzene ring were detected at 6.63 and 7.85 ppm. Two different signals were observed because of the presence of two different substituents in the benzene ring, namely -NH2, and -CO-O-C2H5. The amine is less electron withdrawing than the ester group, which makes the nearby protons slightly more shielded, pushing their signals upfield. Both types of benzene protons appear as duplets due to coupling with a nearby proton in the benzene ring (Analysis based on (Claridge, 2016)).
13C NMR Spectroscopy analysis
Benzocaine 1H NMR data was generated at 600 MHz, but the same equipment can be used to collect the 13C NMR data if the operational frequency is changed to 100 MHz. The change in frequency is necessary because 13C resonates at 1/6 of the 1H frequency (Claridge, 2016). The 13C NMR data of the analysed compound is presented in the figure below:
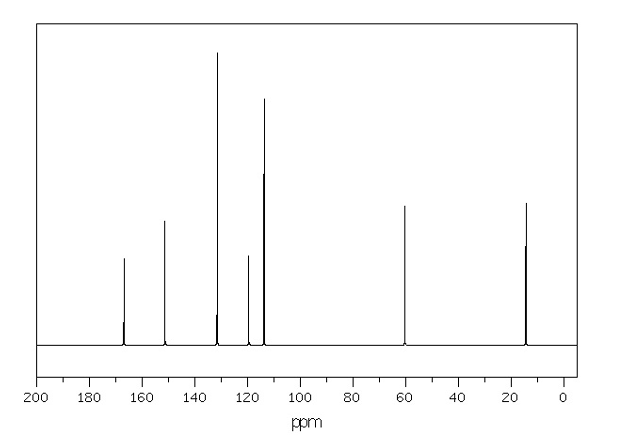
Figure 6: Benzocaine 13C NMR peak assignment
The indicated in the figure above peaks were assigned to benzocaine in the following way:
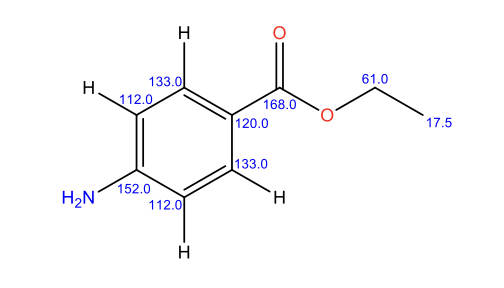
Figure 7: Benzocaine 13C NMR peak assignment
Analysing the 13C NMR experimental data, it is clear that in contrast to 1H NMR, no carbon-carbon coupling is detected. This observation is associated with the fact that the presence of 13C in nature is considerably lower than 1H hydrogen. Similarly to 1H NMR, the corresponding signals appear based on shielding of carbon atoms. Ethyl carbons are the most heavily shielded, which results in signals appearing upfield. In turn, aromatic carbon atoms are less shielded due to the presence of delocalised double bonds, which shifts their signals downfield. Finally, ethyl carbon is the least shielded as a result of electron-withdrawing effects by two oxygen atoms and an adjacent aromatic ring (Analysis based on (Claridge, 2016)).
IR (Infrared) Spectroscopy
To conclude, benzidine characterisation IR data was recorded using a KBr disk. The respective experimental data is presented in Figure 8 below:
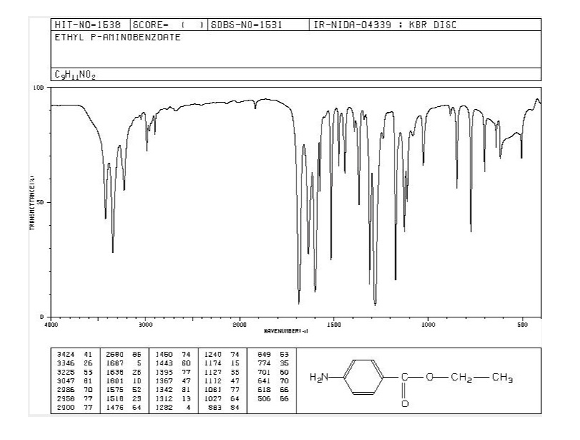
Figure 7: Benzocaine IR (Infrared) data recorded using a KBr disk
In the IR data above, a range of peaks were observed in the 3000-4000 cm-1 region. Correlating with the structure of the molecule, formation of the indicated peaks is associated with the presence of the -NH2 group. In turn, the ester group is detected at 1750-1735 cm-1 as the C=O stretch. At the same time, C-O stretch signal is detected at 1050-1150 cm-1. Signals below 1000 cm-1 correspond to the fingerprint region that can be used for comparison purposes with IR databases (Analysis based on (Dewan, 2019)).
Summary and conclusions
Benzocaine is a highly important drug that has a range of applications in modern medicine. Its synthesis and purification are straightforward, adding to its quality as a prospective drug. By using a combination of MS, IR, 1H, and 13C NMR it was possible to confirm the formation of benzocaine as the main product. Comparing the indicated methods, it is clear that 1H NMR is the most powerful one, as it provides information on the arrangement of hydrogens. This arrangement is further confirmed by 13C NMR, with final molecular mass confirmation by MS and identification of the most important functional groups by IR.
Reference list
Chrubasik, S., Beime, B. & Magora, F., 2012. Efficacy of a benzocaine lozenge in the treatment of uncomplicated sore throat. European Archives of Oto-Rhino-Laryngology, 269(2), pp. 571-577.
Claridge, T., 2016. High-Resolution NMR Techniques in Organic Chemistry. 3rd ed. London: Elsevier Science.
Clayden, J., Greeves, N. & Warren, S., 2012. Organic Chemistry. 1st ed. Oxford: Oxford University Press.
Dewan, S., 2019. Organic Spectrospoy NMR IR Mass and UV: (NMR, IR, Mass and UV). 1st ed. London: CBS Publishing.
DrugBank, 2024. Benzocaine. [Online]
Available at: https://go.drugbank.com/drugs/DB01086
[Accessed 12 September 2024].
Gondim, D., Montagner, A., Pita-Neto, I., Bringel, R., Sandrini, F., Moreno, E., de Sousa, A. & Correia, A., 2018. Comparative Analysis of the Effectiveness of the Topical Administration of Benzocaine and EMLA® on Oral Pain and Tactile Sensitivity. International Journal of Dentistry, 2018(7916274), pp. 1-15.
Lirk, P., Picardi, S. & Hollmann, M., 2014. Local anaesthetics: 10 essentials. European Journal of Anaesthesiology, 31(11), pp. 575-585.
Saadat, M., Banihashemi, K. & Arabani, H., 2023. Synthesis of Benzocaine Analogues via Esterification Reaction in the Presence of Sustainable Natural Deep Eutectic Solvents/Catalyst. Journal of Synthetic Chemistry, 2(August), pp. 148-155.
Singh, R., Patel, P. & Khalili, Y., 2024. Benzocaine, London: StatPearls.